Our water permeation movie and the 2003
Nobel prize for Chemistry
Aquaporins are membrane water channels that play critical roles
in controlling the water contents of cells. These channels are widely
distributed in all kingdoms of life, including bacteria, plants, and
mammals. More than ten different aquaporins have been found in human
body, and several diseases, such as congenital cataracts and
nephrogenic diabetes insipidus, are connected to the impaired function
of these channels. They form tetramers in the cell membrane, and
facilitate the transport of water and, in some cases, other small
solutes across the membrane. However, the water pores are completely
impermeable to charged species, such as protons, a remarkable property
that is critical for the conservation of membrane's electrochemical
potential, but paradoxical at the same time, since protons can usually
be transported readily through water molecules. The results of our
simulations have now provided new insight into the mechanism
underlying this fascinating property. Water molecules passing the
channel are forced, by the protein's electrostatic forces, to flip at
the center of the channel (see the animation), thereby breaking the
alternative donor-acceptor arrangement that is necessary for proton
translocation (read the complete story in our Science
paper). |
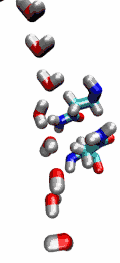
Made with VMD.
Download a larger movie of water
permeation through aquaporins with more structural details
(13MB) |
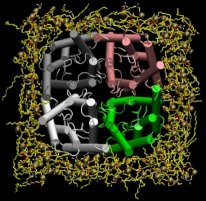 |
The group's recent studies have revealed several structural and
functional features of aquaporins. Our first set of molecular
dynamics (MD) simulations was performed on a fully hydrated model of
aquaporin-1 (AQP1) in a membrane which was based on the electron
microscopy structures. The calculations described the formation of a
water single file inside the channel and revealed the critical role of
the NPA motifs in the stability and function of the channel (Zhu, et al., FEBS
Lett., 2001). The simulations clearly showed the sensitivity of
the results to a proper description of hydrogen bonds between the
asparagine residues in the NPA motifs and the necessity for a higher
resolution structure. |
In the year 2000, Stroud's lab in UCSF succeeded in solving the first high
resolution structure of an aquaporin by x-ray crystallography. The
studied structure was the one of the E. coli glycerol uptake
facilitator (GlpF), which is an aquaglyceroporin, i.e., the
channel is also permeable to small linear sugar molecules such as
glycerol. Nanosecond MD simulations of tetrameric GlpF in a hydrated
patch of POPE lipid bilayer characterized the complete pathway of
substrate conduction in the channel. Analysis of hydrogen bond
interactions of the substrate with the interior of the channel also
explained for the first time why these channels incorporate in their
architecture two characteristic loops, including energetically
unfavorable secondary structure elements, which are conserved in the
whole aquaporin family (Jensen et al.,
Structure, 2001). |
|
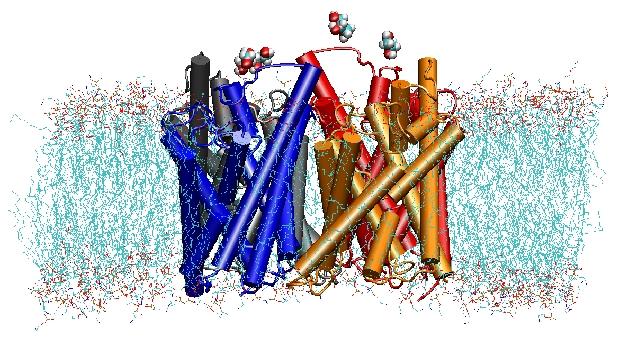
Then, we explored the energetics of the transport event to understand
what barriers a substrate needs to overcome during its passage through
the channel. In order to describe the free energy profile, we
calculated the potential of mean force for the complete conduction
event from the trajectories in which the movement of the substrate
along through the channel was accelerated by applying external forces.
This technique, known as steered
molecular dynamics (SMD), allows the researchers to observe events
within the accessible time scale of the simulations. However, due to
the non-equilibrium nature of the trajectories, in analyzing results
one faces the problem of irreversible work that has to be discounted.
Using the recently discovered equality between the free energy and
work in non-equilibrium systems, Jarzinsky's identity, we could
completely describe the energetics of substrate transport through the
channel. The potential of mean force captures major binding sites and
barriers in the channel is close agreement with the results of MD
simulations and the positions found in the crystal structure.
Moreover, it displays a pronounced asymmetry in its shape, suggesting
that the asymmetric structure of the protein may be functionally
important for an efficient uptake of nutrient molecules from the
environment. (Jensen, et al.,
PNAS, 2002).
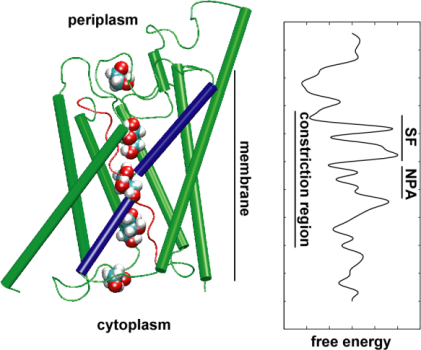 |
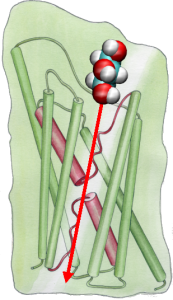 |
Can we simulate the conduction the same way as in experiments?
In order to directly compare the results of MD simulations with biochemical
measurements of the conductivity of membrane water channels, in which
osmotic pressure gradients are used to induce the flow of water across
the membrane, we developed a new methodology for MD simulations. By
applying small forces on water molecules in the bulk region (see
figure), a hydrostatic pressure gradient is generated across the
membrane. The pressure gradient induces conduction rates that can be
studied with MD simulations, which are usually limited to a few
nanoseconds. The method has been applied in the simulation of water
permeation through the GlpF, and has resulted in a linear correlation
between the applied pressure and the flux (Zhu et al.,
Biophys. J., 2002). |
|
Aquaporin research in the news:
|
Aquaporin team:
|
Publications:
Molecular Basis of Proton Blockage in Aquaporins.
Nilmadhab Chakrabarti, Emad Tajkhorshid, Benoît Roux, and Régis Pomès.
Structure, 12: 65-74, 2004.
The mechanism of proton exclusion in aquaporin channels.
Boaz Ilan, Emad Tajkhorshid, Klaus Schulten, and Gregory A. Voth. PROTEINS: Structure, Function, and Bioinformatics, 55:223-228, 2004.
Theory and simulation of water permeation in aquaporin-1.
Fangqiang Zhu, Emad Tajkhorshid, and Klaus Schulten. Biophysical Journal, 86:50-57, 2004.
Electrostatic tuning of permeation and selectivity in aquaporin water channels.
Morten Ø. Jensen, Emad Tajkhorshid, and Klaus Schulten. Biophysical Journal, 85:2884-2899, 2003.
Water and proton conduction through carbon nanotubes as models for biological channels.
Fangqiang Zhu and Klaus Schulten. Biophysical Journal, 85:236-244, 2003.
Glycerol conductance and physical asymmetry of the Escherichia coli glycerol facilitator GlpF.
Deyu Lu, Paul Grayson, and Klaus Schulten. Biophysical Journal, 85:2977-2987, 2003.
Mechanisms of selectivity in channels and enzymes studied with interactive molecular dynamics.
Paul Grayson, Emad Tajkhorshid, and Klaus Schulten. Biophysical Journal, 85:36-48, 2003.
Control of the selectivity of the aquaporin water channel family by global orientational tuning.
Emad Tajkhorshid, Peter Nollert, Morten Ø. Jensen, Larry J. W. Miercke, Joseph O'Connell, Robert M. Stroud, and Klaus Schulten. Science, 296:525-530, 2002.
Energetics of glycerol conduction through aquaglyceroporin GlpF.
Morten Ø. Jensen, Sanghyun Park, Emad Tajkhorshid, and Klaus Schulten. Proceedings of the National Academy of Sciences, USA, 99:6731-6736, 2002.
Pressure-induced water transport in membrane channels studied by molecular dynamics.
Fangqiang Zhu, Emad Tajkhorshid, and Klaus Schulten. Biophysical Journal, 83:154-160, 2002.
The mechanism of glycerol conduction in aquaglyceroporins.
Morten Ø. Jensen, Emad Tajkhorshid, and Klaus Schulten. Structure, 9:1083-1093, 2001.
Molecular dynamics study of aquaporin-1 water channel in a lipid bilayer.
Fangqiang Zhu, Emad Tajkhorshid, and Klaus Schulten. FEBS Letters, 504:212-218, 2001.
Last updated: Dec 29, 2003 by
Emad Tajkhorshid
|